Last Updated on August 29, 2024 by Max
Albert Einstein is often celebrated for his theory of relativity, but his impact on quantum mechanics is equally profound and transformative. While Einstein’s contributions span various areas of physics, his work in quantum mechanics laid the foundation for several crucial developments in the field.
Let’s explore the key contributions of Einstein to quantum mechanics, highlighting his scientific insights, theories, and debates that shaped the evolution of this field.
The Photoelectric Effect (1905)
One of Einstein’s most famous contributions to quantum mechanics is his explanation of the photoelectric effect, for which he was awarded the Nobel Prize in Physics in 1921. In 1905, Einstein proposed that light consists of discrete packets of energy called “quanta” or “photons.”
- Key Insight: When light shines on a material, it releases electrons from its surface. According to classical wave theory, the energy of the emitted electrons should depend on the light’s intensity. However, experiments showed that electron energy depends on the light’s frequency, not its intensity.
- Einstein’s Explanation: Einstein suggested that light quanta (photons) interact with electrons like particles. Each photon has an energy proportional to its frequency, given by \(E = h \nu\), where \(h\) is Planck’s constant, and \(\nu\) is the frequency. If the energy of a photon exceeds the material’s work function, it can eject an electron. This idea fundamentally challenged the classical wave theory of light and supported Max Planck’s quantum hypothesis.
Quantum Theory of Specific Heat (1907)
Einstein extended quantum theory to explain the specific heat of solids, addressing a problem that classical physics could not solve. Classical physics predicted that the specific heat capacity of solids should be constant at all temperatures, which contradicted experimental observations.
- Einstein’s Model: He applied Planck’s quantum hypothesis to the vibrations of atoms in a solid lattice, treating each vibrational mode as a quantum harmonic oscillator with discrete energy levels. According to this model, the specific heat should decrease at low temperatures, which aligned with experimental data.
- Impact: This was one of the first applications of quantum theory to describe matter. It paved the way for the development of quantum mechanics in solid-state physics and the formulation of quantum statistics.
Stimulated Emission and the Foundation for Lasers (1917)
Einstein introduced the concept of stimulated emission in 1917, which later became the foundation for developing lasers.
- Theory of Radiation: Einstein developed a theory describing the absorption, spontaneous emission, and stimulated emission of radiation by atoms. He derived the coefficients (now known as Einstein A and B coefficients) that quantify the probability of each process occurring.
- Stimulated Emission: He postulated that when an atom in an excited state interacts with an electromagnetic wave of a specific frequency, it can emit a photon identical in phase, direction, and energy to the incident wave. This idea of stimulated emission is the core principle behind laser operation.
Bose-Einstein Statistics and Condensation (1924-1925)
In collaboration with the Indian physicist Satyendra Nath Bose, Einstein developed a statistical framework for a new type of particle indistinguishability, leading to Bose-Einstein statistics.
- Bose-Einstein Condensate (BEC): Einstein extended Bose’s work on photons to atoms, predicting that at ultra-low temperatures, a large fraction of bosons would occupy the lowest quantum state, forming a new state of matter called a Bose-Einstein Condensate.
- Impact on Quantum Theory: This work introduced the concept of quantum statistics and laid the groundwork for quantum field theory. The experimental realization of BEC in 1995 provided evidence for Einstein’s predictions and opened new avenues in quantum physics and condensed matter research.
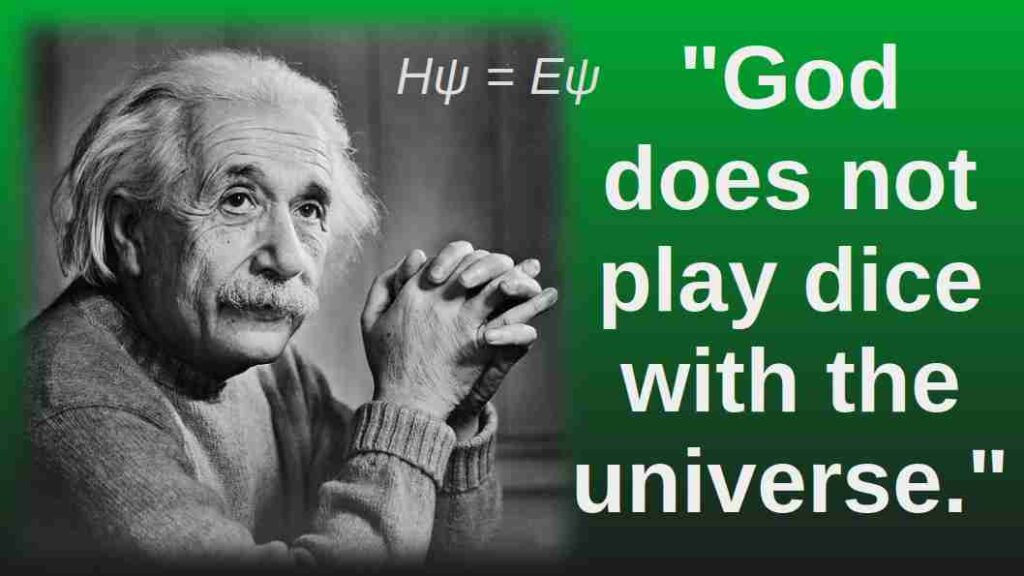
Quantum Entanglement and the EPR Paradox (1935)
Einstein, along with Boris Podolsky and Nathan Rosen, proposed the EPR paradox, challenging the completeness of quantum mechanics.
- EPR Paper: In 1935 [1], Einstein and his colleagues published a paper titled “Can Quantum-Mechanical Description of Physical Reality Be Considered Complete?” They argued that quantum mechanics is not a complete theory because it allows for “spooky action at a distance,” where entangled particles instantaneously affect each other, violating locality and determinism.
- Einstein’s Objections: Einstein was uncomfortable with the probabilistic interpretation of quantum mechanics and the idea of non-locality. He believed in a deterministic universe and sought a hidden variable theory to restore causality and locality. Despite being a critic, Einstein’s arguments spurred significant debates that led to deeper understandings and interpretations of quantum mechanics.
- Impact on Quantum Theory: The EPR paradox led physicists like John Bell to study the nature of quantum entanglement. This investigation resulted in Bell’s Theorem in 1964. The theorem showed that no local hidden variable theory could reproduce all the predictions of quantum mechanics. This paved the way for experimental tests of quantum entanglement and the development of quantum information theory.
Einstein’s Contributions to Wave-Particle Duality
While Einstein was not the first to propose the wave-particle duality, his work on the photoelectric effect and other phenomena helped establish the dual nature of light and matter.
- Einstein’s Perspective: Einstein believed in a deeper underlying reality beyond the duality, hinting at the existence of a unified theory that would reconcile wave and particle descriptions.
- Influence on Quantum Mechanics: His questioning of the wave-particle duality and pursuit of a unified theory inspired later developments in quantum mechanics, encouraging physicists to explore the fundamental nature of reality.
Quantum Theory and the Cosmological Constant (1917)
Einstein introduced the cosmological constant in his equations for General Relativity, which he later referred to as his “greatest blunder.” However, this constant is crucial in understanding quantum mechanics and cosmology today.
- Vacuum Energy and Quantum Fluctuations: The cosmological constant is related to the energy density of the vacuum, a concept tied to quantum field theory and the Casimir effect. Modern quantum mechanics and cosmology see the cosmological constant as a manifestation of quantum vacuum fluctuations, contributing to the accelerating expansion of the universe.
Einstein’s Dual Role in Quantum Mechanics
Einstein’s relationship with quantum mechanics was complex. He was both a pioneer and a skeptic. While he made groundbreaking contributions to its development, he also famously challenged its completeness and interpretations, advocating for a deterministic view of nature.
- Legacy: Einstein’s debates with Niels Bohr and his critiques of quantum mechanics have shaped the philosophical foundations of quantum theory. His insistence on rigor and clarity continues to influence discussions on the interpretation of quantum mechanics, contributing to its growth as a robust scientific discipline.
Conclusion
Albert Einstein made pivotal contributions to quantum mechanics. He helped establish many foundational principles, from the quantization of light to the statistical mechanics of particles and the study of quantum entanglement. His challenges to the interpretation of quantum mechanics have spurred decades of research, experimentation, and debate. This underscores his lasting impact on the field.
Einstein’s relationship with quantum mechanics involved both contributions and criticisms. Yet, his influence remains profound, shaping our understanding of the quantum world and guiding future discoveries. His work embodies the spirit of scientific inquiry and the pursuit of truth. This makes him one of the most remarkable figures in the history of quantum physics.
References
[1] Einstein, A., Podolsky, B. and Rosen, N., 1935. Can a quantum-mechanical description of physical reality be considered complete?. Physical Review, 47 (10), p.777.

I am a science enthusiast and writer, specializing in matter-wave optics and related technologies. My goal is to promote awareness and understanding of these advanced fields among students and the general public.