Last Updated on August 31, 2024 by Narayan
Niels Bohr, a Danish physicist, is one of the most influential figures in the development of quantum mechanics. His work laid the foundation for much of our current understanding of atomic and subatomic processes.
Bohr’s contributions to quantum mechanics are multifaceted, involving theoretical advancements, philosophical insights, and foundational principles that continue to guide quantum research today.
This article explores Bohr’s most significant contributions to quantum mechanics and their lasting impact on science.
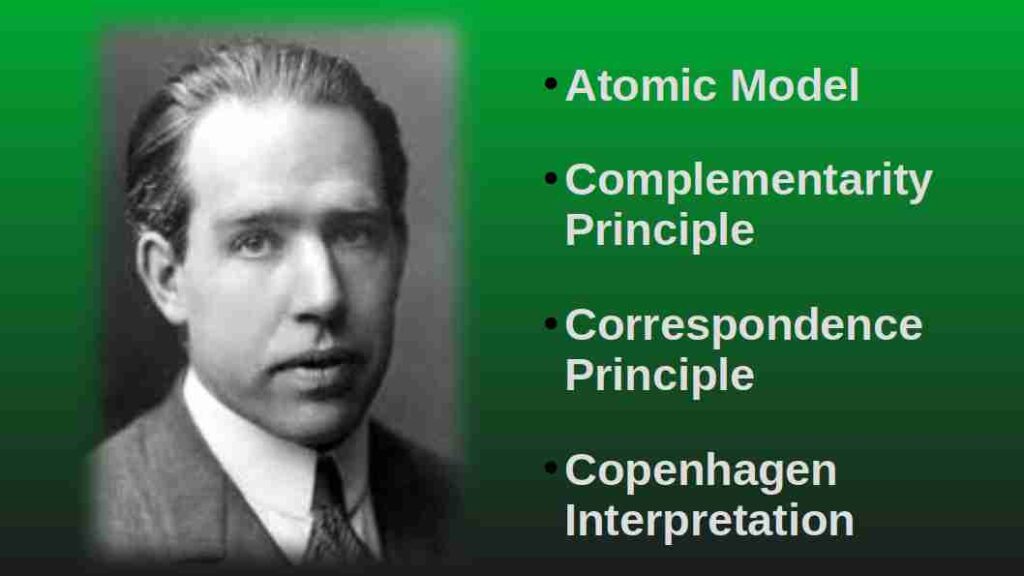
Bohr’s Atomic Model: The Quantum Leap
One of Bohr’s earliest and most groundbreaking contributions was his atomic model, proposed in 1913. The model was developed to explain the stability of atoms and the discrete lines observed in the emission spectra of hydrogen.
Before Bohr, the classical Rutherford model of the atom, which consisted of electrons orbiting a central nucleus, could not explain why atoms do not collapse under electrostatic attraction or why they emit light at specific frequencies.
Bohr introduced the idea that electrons exist in discrete orbits around the nucleus and can only occupy specific energy levels. Here are the key features of his model:
- Quantization of Orbits: Bohr postulated that electrons move in fixed orbits around the nucleus without radiating energy. These orbits correspond to specific energy levels, and an electron can only occupy a certain level without falling into the nucleus.
- Energy Transitions and Quantum Jumps: Bohr’s model explained that electrons could transition between these quantized orbits by absorbing or emitting photons, corresponding to the energy difference between two levels. This concept accounted for the discrete spectral lines of hydrogen observed experimentally.
- Bohr’s Quantization Rule: Bohr introduced the quantization condition for angular momentum, stating that the angular momentum of an electron in orbit is an integer multiple of \( \hbar = \frac{h}{2\pi} \), where \( h \) is Planck’s constant. This was a radical departure from classical mechanics.
Bohr’s atomic model, while eventually superseded by the more comprehensive quantum mechanical models, marked a pivotal step in the transition from classical to quantum physics. It introduced the concept of quantization and helped establish quantum theory’s probabilistic nature.
Complementarity Principle
Bohr’s complementarity principle, introduced in 1927, became a cornerstone of quantum mechanics.
The principle asserts that quantum objects, like electrons and photons, exhibit both particle-like and wave-like properties, but these properties cannot be observed simultaneously. The nature of what is observed depends on the experimental setup and the type of measurement performed.
Key aspects of the complementarity principle include:
- Wave-Particle Duality: According to Bohr, the wave and particle descriptions are complementary; they represent different perspectives of the same reality. A quantum entity will behave as a particle or a wave, depending on how it is measured.
- Measurement and Observation: Bohr emphasized that the act of measurement affects the system being observed. This idea challenged classical notions of objective reality and contributed to the development of the Copenhagen interpretation of quantum mechanics.
- Philosophical Implications: Complementarity extended beyond physics, influencing discussions in philosophy, biology, psychology, and other disciplines. Bohr argued that complementary views are essential for a holistic understanding of complex systems.
The Correspondence Principle: Connecting Classical and Quantum Worlds
The correspondence principle, formulated by Bohr in the early 1920s, provided a bridge between quantum mechanics and classical physics. It states that the behavior of quantum systems should converge to classical mechanics in the limit of large quantum numbers or macroscopic scales. This principle ensured that quantum mechanics did not contradict classical mechanics but extended it to the atomic and subatomic worlds.
The correspondence principle had several significant implications:
- Consistency with Classical Physics: It provided a theoretical foundation for quantum mechanics, demonstrating that it could reproduce classical results in appropriate limits.
- Guiding the Development of Quantum Theory: Bohr used the correspondence principle to derive quantum rules and relations, particularly for complex systems where direct quantum solutions were challenging.
Copenhagen Interpretation
Niels Bohr was a central figure in developing the Copenhagen interpretation of quantum mechanics, which he formulated in collaboration with Werner Heisenberg and other physicists in the 1920s. This interpretation remains one of the most widely accepted explanations of quantum mechanics.
Key elements of the Copenhagen interpretation include:
- Wave Function and Probability: The wave function, denoted by \( \psi \), represents the probability amplitude of finding a particle in a given state. The square of the wave function’s absolute value, \( |\psi|^2 \), provides the probability density for various measurement outcomes.
- Uncertainty Principle: Heisenberg’s uncertainty principle, closely associated with Bohr’s ideas, states that certain pairs of physical properties, such as position and momentum, cannot be simultaneously measured with arbitrary precision. Bohr emphasized that this principle reflects fundamental limits of nature, not measurement shortcomings.
- Observer Effect: The act of observation plays a crucial role in determining the outcome of quantum measurements. The Copenhagen interpretation suggests that the physical state of a quantum system is not determined until it is observed, leading to the concept of wave function collapse.
Bohr-Einstein Debates
Bohr’s debates with Albert Einstein, particularly during the Solvay Conferences of the 1920s and 1930s, were critical in shaping the philosophical foundations of quantum mechanics. Einstein famously challenged the probabilistic nature of quantum theory, expressing skepticism with his statement, “God does not play dice with the universe.”
Bohr defended the Copenhagen interpretation, arguing that quantum mechanics provides a complete description of nature, even if it is inherently probabilistic. These debates addressed core questions about reality, determinism, and the role of the observer in quantum theory.
Though neither physicist convinced the other, their discussions stimulated critical thinking and contributed significantly to the development of quantum mechanics.
Contributions to Quantum Field Theory
While Bohr is primarily known for his work on atomic structure and the philosophical foundations of quantum mechanics, he also made notable contributions to quantum field theory.
Bohr collaborated with colleagues like Léon Rosenfeld to address the problem of measuring electromagnetic fields in quantum mechanics. Their work laid the groundwork for understanding field quantization and the interaction of matter with radiation.
Bohr’s Legacy in Quantum Mechanics
Niels Bohr’s contributions extend beyond his scientific theories. He played a pivotal role in mentoring a generation of physicists, including Heisenberg, Pauli, Dirac, and many others, who became leading figures in quantum mechanics. Bohr’s institute in Copenhagen became a hub for research, fostering collaboration and the exchange of ideas that shaped modern physics.
Bohr’s emphasis on the complementarity principle and the Copenhagen interpretation also had far-reaching implications for the philosophy of science. His ideas about the limits of knowledge, the nature of reality, and the role of observation have influenced not only quantum mechanics but also fields like cognitive science, biology, and philosophy.
Conclusion
Niels Bohr was a key figure in quantum mechanics, offering both theoretical ideas and philosophical insights. His atomic model introduced the concept of quantized energy levels. The complementarity principle reshaped our understanding of reality, and his work on the Copenhagen interpretation set a new standard for quantum theory. Through his debates, guidance, and leadership, Bohr helped build the foundation for a major scientific revolution in the 20th century.
Bohr’s work is still crucial to our study of quantum phenomena. It shows that understanding the mysteries of the quantum world involves both scientific investigation and philosophical thinking.
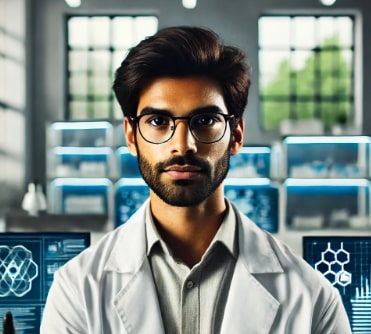
As a researcher specializing in matter-wave optics and numerical methods, I am passionate about demystifying complex concepts in quantum mechanics. My aim is to make these advanced topics accessible and understandable for all.